Headline:
Methane Cracking – a Promising Bridge Technology on our Road to Decarbonization
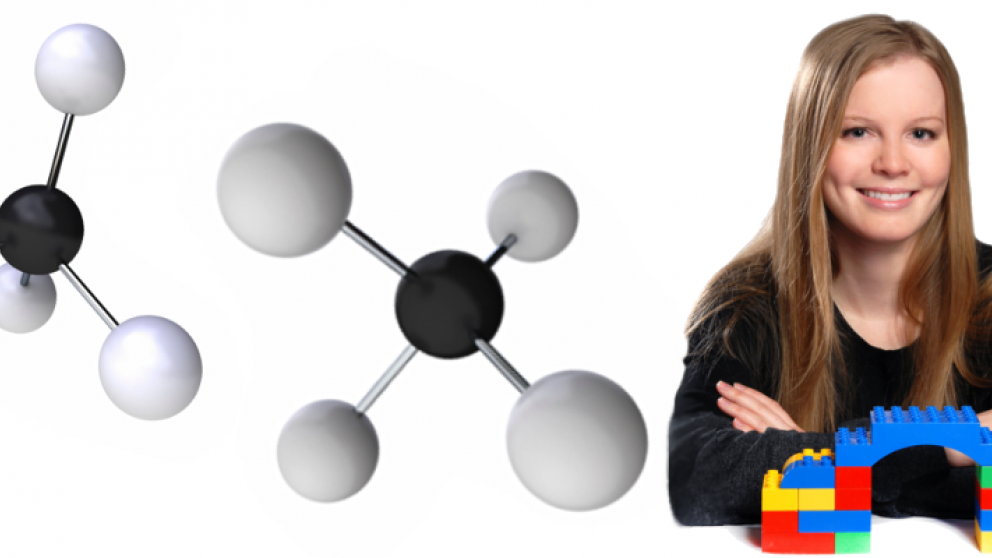
Meeting global energy needs represents a key challenge for climate change mitigation. This is because the energy system today is dominated by fossil fuels, which emit a significant amount of CO2 to the atmosphere. In fact, the energy sector is responsible for the majority of anthropogenic emissions, contributing more than 75 per cent of global greenhouse gas emissions.[1] In order to avoid dangerous climate change it is crucial that we transition our global energy system away from fossil fuels and towards low-carbon, sustainable energy. Of course, it is an immense challenge to make this transition. Bridge technologies, in which a cleaner fossil fuel technology replaces a dirtier one, offer a realistic approach to this transition.
The logic behind bridge technologies is that it is unrealistic to immediately cease all fossil fuel use. If fossil fuels will continue to be used in the short-term, then they should be used in a pragmatic way that reduces greenhouse gas and pollutant emissions. This will mitigate the extent of climate impact from fossil fuels until it becomes economically feasible to completely switch from fossil fuels to renewables. Moreover, if bridge technologies can facilitate the development of sustainable energy infrastructure that can continue to be used after individual bridge technologies have been phased out, this will further contribute to climate change mitigation.
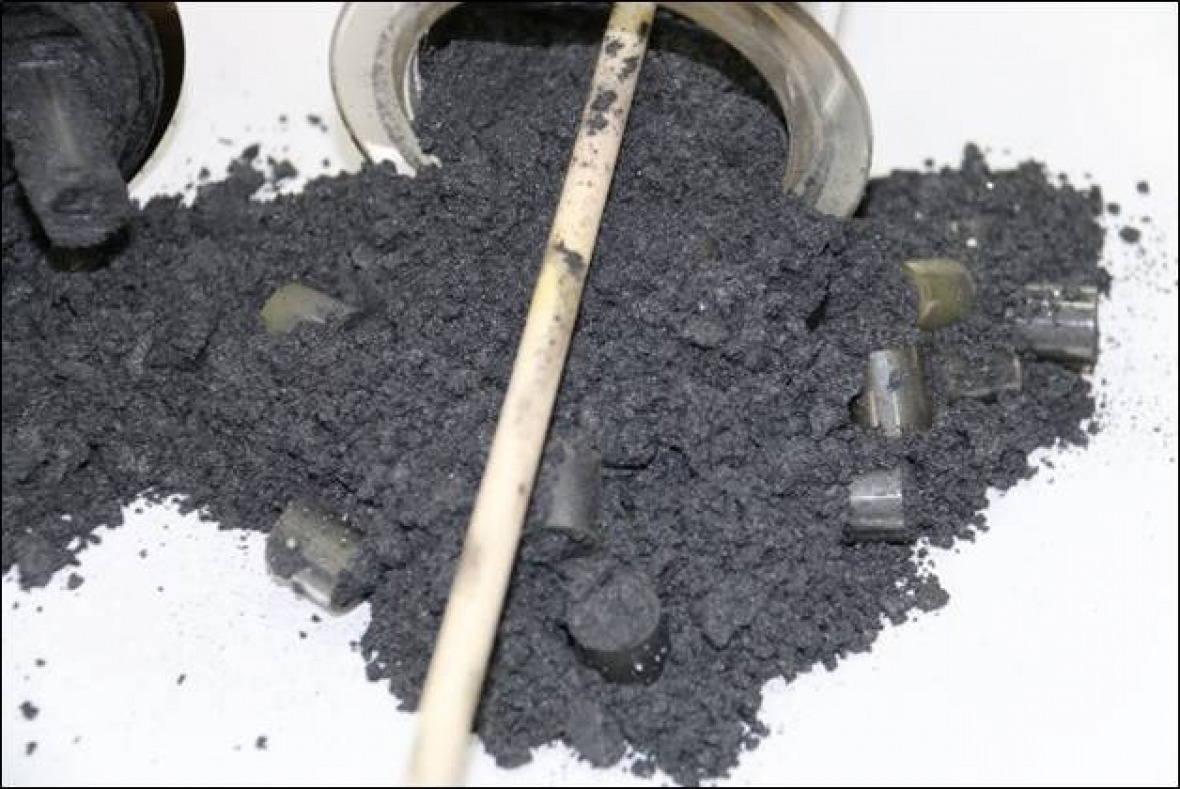
Methane cracking is a promising new method that may serve this purpose. In this method, methane is separated at high temperatures into its molecular components – hydrogen and pure carbon (see below). Importantly, because it is carried out in an oxygen-free environment, the reaction itself generates zero emissions of CO2. The hydrogen produced serves as an energy carrier, while the carbon may be an asset for use in commercial applications such as the production of carbon fiber. Alternatively, it can be easily stored for future use. Recent research indicates that methane cracking is a viable process, with our latest, as yet unpublished, findings showing that this technology may well be suitable for industrial scale application.
CH4 -> C + 2 H2 |
Hydrogen has great potential as a sustainable fuel. It has a high energy density by weight, and simply forms water upon generation of electricity or combustion. Because it is not a greenhouse gas and does not generate long-lived greenhouse gases when used for energy, and because it can be produced from sustainable energy sources (e.g., electrolysis facilitated by renewable energy), hydrogen has the potential to reduce emissions of greenhouse gases in the energy sector. Additionally, the hydrogen fuel cell possesses a high energy efficiency compared to the internal combustion engine, making hydrogen especially attractive as a way to reduce emissions in the transportation sector.
Due to the many advantages of hydrogen, the ‘hydrogen economy’ has been proposed in which hydrogen serves as one of the main energy carriers to satisfy global energy needs. While the hydrogen economy received extensive attention by the media and heavy investment in its research by the United States government in the early 2000s, the hype eventually subsided and the bulk of funding in the USA has since been diverted to electric vehicles. This turnabout was driven by challenges faced by the hydrogen economy relating to the transportation, distribution and storage of hydrogen as a result of its low energy density by volume. But regardless of their current popularity, it is not clear that electric vehicles are the best or only solution in a low-carbon, sustainable energy future. Many different energy forms will likely be part of the energy mix, and the specific choice will depend on the application. In fact, Toyota introduced one of the first commercially-available hydrogen fuel cell vehicles, the Toyota Mirai, in the USA and Europe in 2015.[2,3]
Despite the promise of methane cracking in reducing CO2 emissions and its potential to foster the development of the infrastructure required in the hydrogen economy, there is another aspect that must be considered in the feasibility of methane cracking as a bridge technology: natural gas. Natural gas supplies methane feedstock to the methane cracking process. Throughout the entire natural gas supply chain, methane leakage occurs. This is problematic because methane is a potent greenhouse gas with a heating ability (i.e., global warming potential) 86 times greater than CO2 over a 20-year period and 34 times over a 100-year period.[4] A review of twenty years of literature on natural gas emissions in the United States and Canada found that atmospheric methane concentrations are much higher than predictions based on current emissions inventories, and part of the discrepancy may be due to the natural gas sector.[4] Recent studies have found that methane leakage from natural gas production may be much higher than current estimates, and may be even greater for shale gas – an unconventional gas form whose production is rapidly expanding in the USA and may well expand abroad.[6,7]
Bridge technologies such as methane cracking have the potential to reduce the warming impact of fossil fuels that will continue to be used in the short-term. In order to understand methane cracking’s feasibility as a bridge technology, a thorough assessment is required. More understanding is needed on the industrial scalability of methane cracking technology, and challenges must be overcome for the hydrogen economy (particularly in the areas of transportation, distribution and storage). Finally, it is important that both CO2 and CH4 emissions are considered in methane cracking’s ability to mitigate the climate warming impact. Importantly, more understanding is needed on actual methane leakage in natural gas production and the potential to mitigate methane emissions from this sector.
- http://www.wri.org/blog/2015/06/infographic-what-do-your-countrys-emissions-look
- http://toyotanews.pressroom.toyota.com/releases/toyota+mirai+owners+jump+future.htm
- https://www.toyota-europe.com/world-of-toyota/articles-news-events/2014/the-toyota-mirai.json
- IPCC, Climate Change 2013: The Physical Science Basis. Contribution of Working Group I to the Fifth Assessment Report of the Intergovernmental Panel on Climate Change, Cambridge University Press. 2013. http://www.climatechange2013.org/images/report/WG1AR5_Chapter08_FINAL.pdf, p. 714
- R. Brandt, G. A. Heath, E. A. Kort, F. O’Sullivan, G. Pétron, S. M. Jordaan, P. Tans, J. Wilcox, A. M. Gopstein, D. Arent, S. Wofsy, N. J. Brown, R. Bradley, G. D. Stucky, D. Eardley and R. Harriss, Science 2014, 343, 733–735.
- W. Howarth, R. Santoro and A. Ingraffea, Clim. Change, 2011, 106, 679-690.
- W. Howarth, Energ. Sci. Eng., 2014, 2, 47-60.
Header image: istock/biosurf (methane molecules); IASS/Photoatelier Pfeil
Add new comment